This post is a summary of the excellent recent paper on the above topic in Economic Geology: Jeffrey W. Hedenquist, Antonio Arribas; Exploration Implications of Multiple Formation Environments of Advanced Argillic Minerals. Economic Geology 2022;; 117 (3): 609–643. https://doi.org/10.5382/econgeo.4880
Introduction
Hydrothermal ore deposits are associated with alteration minerals, and one such alteration type is “advanced argillic,” found in relatively shallow geological environments where minerals like alunite, kaolinite, dickite, and pyrophyllite indicate the presence of reactive fluids. This term encompasses a range of minerals, including sericite, quartz, alunite, pyrite, tourmaline, topaz, and more. Some of these minerals are shared with other alteration types, like the kaolinite, dickite, and halloysite clays of the argillic term.
Recognition and interpretation of advanced argillic minerals have increased during mineral exploration in recent decades, facilitated by SWIR spectrometry. This extensive mineralogical information provides insights into the formation environment and its relation to potential mineral deposits.
Understanding advanced argillic and related alteration types is essential for mineral exploration and assessment. The provided framework guides exploration efforts and the study of alteration mineralogy across a wide range of hydrothermal settings, from subaerial to submarine, and varying depths and temperatures.
Executive Summary
- Advanced argillic minerals include alunite, anhydrite, aluminosilicates (kaolinite, halloysite, dickite, pyrophyllite, andalusite, zunyite, and topaz), and diaspore. Advanced argillic minerals are key indicators of specific geological alteration environments and are commonly associated with hydrothermal systems and volcanic activity.
- The formation of these minerals is closely tied to the pH levels, depths, and geochemical conditions of their respective environments.
- These minerals form in five distinct geologic environments of hydrolytic alteration, with pH ranging from 4 to less than 1, often at depths below 500 meters.
- Formation environments which range from high-temperature volcanic vapor condensation to supergene weathering processes include:
- Intrusion-related hydrothermal systems evolving to white-mica stability, leading to pyrophyllite and dickite formation.
- Vapor condensation near volcanic vents, resulting in alunite and kaolinite formation.
- Boiling of hydrothermal liquid generating vapor with CO2 and H2S, leading to the formation of kaolinite and alunite.
- Condensation of vapor below the water table, causing intermediate argillic alteration of clays, kaolinite, and Fe-Mn carbonates.
- Supergene alteration due to post-hydrothermal weathering and oxidation, forming kaolinite, alunite, and Fe oxyhydroxides.
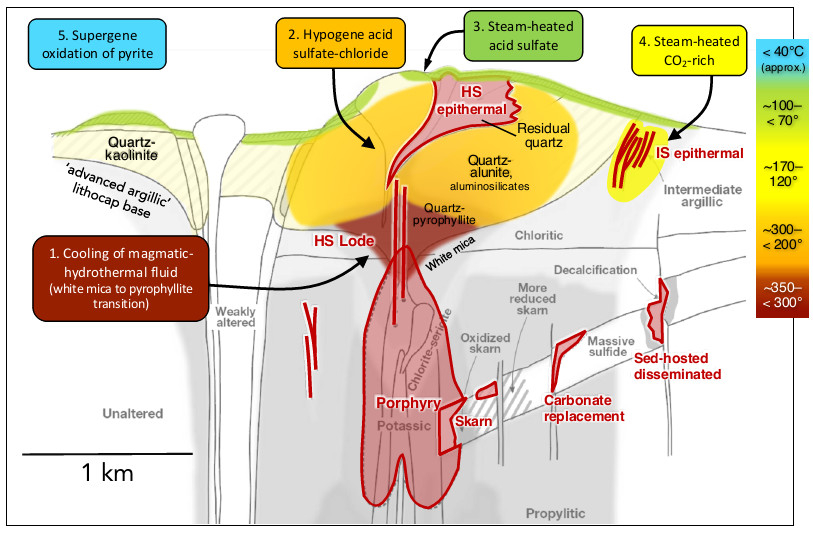
- High solubility of Al and Fe hydroxides at pH <2 results in rock dissolution except for SiO2, which remains as siliceous residue (residual or vuggy silica) generating the often conspicuous and extensive lithocaps.
- Condensation of vapor below the water table results in a pH of 4–5 due to the formation of carbonic acid (H2CO3). This condensate acts as a diluent for the ascending parental NaCl liquid, leading to intermediate argillic alteration with clays, kaolinite, and Fe-Mn carbonates.
- Boiling of hydrothermal liquid generates vapor with CO2 and H2S, causing oxidation of H2S to form a steam-heated acid-sulfate solution with a pH of 2–3. In this environment, kaolinite and alunite form above the water table at temperatures below 100°C.
- Alunite and kaolinite which form from vapor condensation near volcanic vents driven by magmatic SO2 and HCl condensation into groundwater, are significant components of advanced argillic alteration and are often used as indicators of valuable mineral deposits like copper and gold. Alunite and kaolinite form a halo around residual quartz, potentially becoming mineralized in lithocaps.
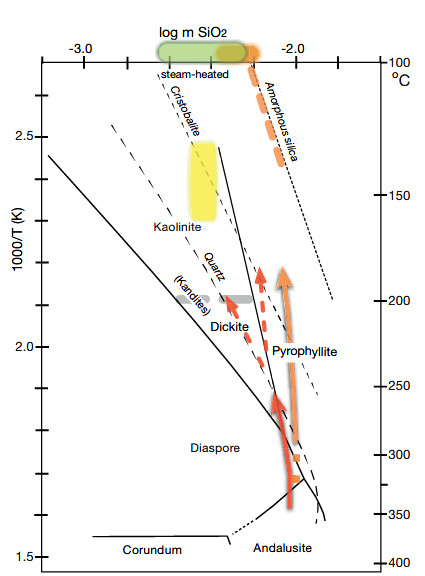
The diagram depicts the relationship between temperature and log m (K/H) based on experimental data from Hemley and Jones (1964), Montoya and Hemley (1975), and Sverjensky et al. (1991). The chart has boundaries at 1 kbar, adjusted for water vapor pressure below 300°C. It uses the K/H ratio ([KCl + K+]/[HCl + H+]). The kaolin group and white-mica fields are divided into dickite and kaolinite, and muscovite and illite, based on temperature stabilities observed in geothermal systems. Different fluid compositions and pathways for four hydrolytic alteration environments ranging from 350°C to 100°C are color-coded. The cooling path of white mica to pyrophyllite is marked with a red arrow. To the left of log m (K/H) = 0, the diagram is schematic, illustrating the relative position of the field of residual quartz, where aluminum hydroxide solubility increases sharply at a pH less than 2.
The composition of acidic springs at White Island and Satsuma Iwojima, which involve both sulfuric and hydrochloric acids, is represented by an orange bar. The residual quartz field and the slope of cooling magmatic vapor condensates (orange arrow) are derived from modeling of Satsuma Iwojima condensates at high water/rock ratio and high SO2/H2S. The slope of the orange arrow will be lower if the solution is closer to SO2 = H2S. Alunite + pyrite are stable at temperatures below 300°C at high sulfate/sulfide ratios.
Steam-heated acid-sulfate springs at Waiotapu and Yellowstone are depicted with a green bar. They have low pH values and contain sulfuric acid, which is locally capable of mobilizing aluminum. The composition of CO2-rich steam-heated fluid sampled from geothermal wells on the margin of the Ohaaki-Broadlands geothermal system is presented in a yellow field, reflecting the reactivity due to carbonic acid and resulting alteration products of clays and kaolinite.
- Supergene alteration is caused by post-hydrothermal weathering and oxidation, producing pH <1 liquid due to high H2SO4 concentrations in the vadose zone.
- The principal features of the five alteration environments examined here, and their implications for exploration, include the following:
- In porphyry environments, pyrophyllite and dickite, sometimes with diaspore, can form at the top, near the transition to the lithocap. This results from the cooling of a white-mica-stable fluid and indicates the presence of a porphyry system.
- Residual quartz, accompanied by quartz-alunite halos and pyrite, develops due to the condensation of hypogene magmatic vapor into groundwater. This zoned hypogene alteration is mostly devoid of metals, with gold and sulfides associated with lithocaps primarily originating from input by a white-mica-stable fluid.
- Alterations due to the release of low-temperature aqueous vapor from boiling CO2- and H2S-bearing liquids result in two environments of hydrolytic alteration. Steam-heated acid-sulfate alteration occurs in the vadose zone, marked by kaolinite and alunite, but is generally not associated with metal anomalies. Steam-heated CO2-rich condensates form below the water table, leading to broad halos of clay alteration, kaolinite, and Fe-Mn carbonate minerals.
- Supergene acidity is generated by the exposure of pyrite to weathering and atmospheric oxidation. In most cases, supergene alteration occurs post-mineralization and results in minerals like kaolinite, porcellaneous alunite, jarosite, Fe oxyhydroxides, and others in various geological environments.
When engaging in geological mapping and logging, it is of paramount importance to discern the significance of a specific group of alteration minerals. This involves providing precise details about the mineralogical composition, the characteristics of the alteration zone, and its relationship with sulfides. The term “advanced argillic” is used to describe a group of minerals, primarily aluminosilicates and sulfates, which form in a diverse array of geological settings. Argillic alteration, which includes minerals like kaolinite, is also part of some advanced argillic assemblages. This complexity underscores the necessity for accurately identifying the minerals present and furnishing detailed descriptions of the alteration occurrences, encompassing both textures and the morphology of the alteration zone and avoiding use of the term “advanced argillic” and other often misused terms like potassic, phyllic, argillic (intermediate argillic), propylitic, and other general categories.
Thanks for the papier